Create a free profile to get unlimited access to exclusive videos, sweepstakes, and more!
How do you feed the insatiable appetite of a nuclear black hole?
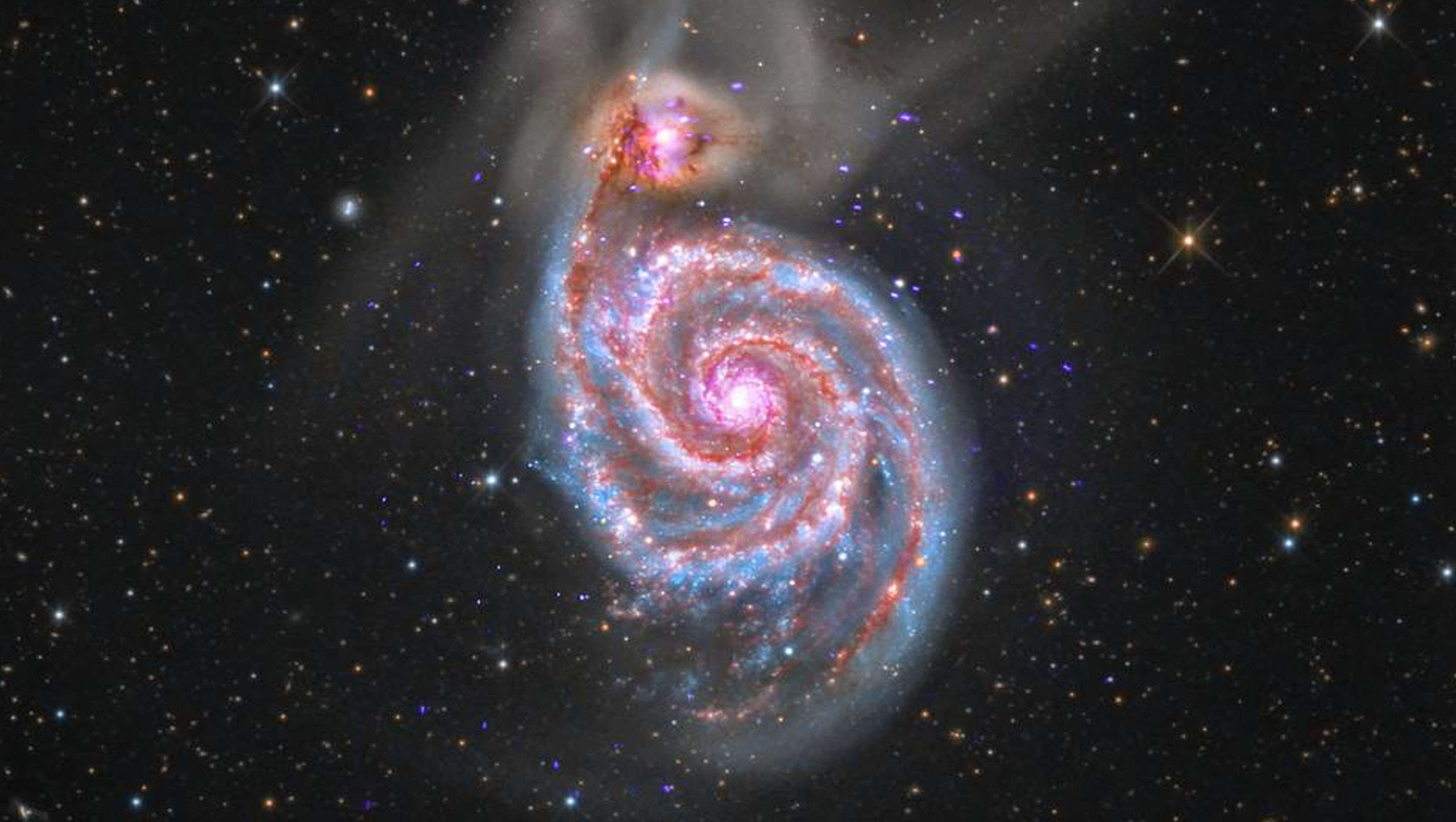
If you were a photon, following the swirl of a spiral galaxy like the Milky Way or M51 (above) would eventually lead you spiraling into doom — the supermassive black hole that is its nucleus. But it gets worse.
Some nuclear black holes in spiral galaxies have an appetite too monstrous for the usual inflow of star stuff that eventually makes it past the event horizon and into their gaping maw. Massive galactic bars, or linear structures of stars and gas that stretch from one arm of a spiral galaxy to the other, are what feed these black holes, as a boom in star formation occurs around them. But what about when you find a spiral galaxy where this is not supposed to happen?
ESO 320-G030 is that problematic galaxy. Some 150,000 light years away from Earth, it has a bar around 60,000 light years long and (because some black holes apparently can never get enough) a second bar a tenth that size which is perpendicular to the larger one.
This is what astronomer Eduardo Gonzalez-Alfonso, who recently co-authored a study in Astrophysics of Galaxies, and his research team decided to investigate. Molecules that were found to exist in the galaxy turned out to be different from the types of molecules expected to be in interstellar space. They modeled the shapes and infrared absorption lines H2O, some of its isotopes and ionized variants, and other types of molecules such as OH (hydroxide), NH (nihonium) and ammonia (NH3), to find out how huge the starburst in ESO 320-G030 was and what was feeding it.
ESO 320-G030 is dusty, and there may be so much dust that it obscures the view of a black hole lurking in its depths, but there is more to this mystery. There wasn’t any previous evidence of a black hole that would scarf down the material in those bars, but this galaxy was still pumping out star after star in its nuclear region without any signs of a nucleus.
“It turns out that H2O (and other molecular species such as OH, CH, and CH+) couples very well with the far-infrared radiation field in the source (emitted by dust grains that are heated by stars and active galactic nuclei) because it has transitions between rotational levels with high transition probabilities and in the far-infrared,” Gonzalez-Alfonso told SYFY WIRE. “This means that H2O is mainly excited by this far-IR radiation field, rather than by collisions, which is very interesting because, probing the radiation field in the source, it is sensitive to the luminosity of the region.”
To model what is otherwise invisible to the human eye, the research team used a software code to figure out excitation — the process in which particles become more energetic after they are fueled with more energy — and the comparable emission and absorption of water as determined by factors such as the amount of H2O, amount and temperature of dust, and size of the region. These factors can then be compared to the emission and absorption that was observed. It was transitions of hydroxide that started to reveal the gas inflow that was sustaining such a yearly nuclear starburst 20 times the mass of the Sun.
“The first indication was that some OH transitions, observed in absorption against the continuum (radiation emitted by dust), showed a line profile that was redshifted relative to the rest of the lines, meaning that the gas responsible for those lines was moving away from the observer and in the direction of the source of far-IR radiation. This was suggesting an inflow,” Gonzalez-Alfonso said. “We know that galaxies at higher redshifts had overall accretion rates from the cosmic web that were similar or even higher than in the local ESO 320-G030.”
Because light can be absorbed, scattered, or reflected by dust depending on what makes up that dust, Gonzalez-Alfonso and his team needed to see beyond it. Hi-res images from ALMA (Atacama Large Millimeter Telescope) observations of ESO 320-G030 revealed even more of what was hiding behind all that dust. These images made it possible to calculate how much of an inflow was headed towards the nuclear region, which also revealed the rate of star formation in that area—which meant this inflow really was behind the nuclear starburst.
The astronomers also discovered more physical things about the galaxy which give it most of its luminosity. Using data from the now-defunct Herschel Space Observatory, they found it to have an envelope of gas and dust some 500 light years across. Gonzalez-Alfonso was also able to see an enormous and really dense disk around the nucleus, which surrounded the core of warm dust that powers the galaxy’s starburst. As gas and dust accumulate, they create a bulge that evolves into a supermassive black hole.
Observed lines of molecules were determined to have certain energy levels that corresponded with these features. For example, high energy levels mean there must be a strong but small field full of hot dust, because that is what it takes to excite the H2O molecules. A much weaker and more extended envelope is inferred if energy levels are low enough.
“We could observe and detect up to 20 lines of H2O, at different energies and with different properties,” he said. “With 20 lines, we arrived at the conclusion that the dust and gas envelope, the circumnuclear disk and the core are required to explain all the available data.”
Not only has ESO 320-G030 been demystified, but it could tell us even more about the distant universe, especially galaxies that are billions of light years away, which means we could be seeing them when they were at their peak of star formation. How galactic bars and gas inflows form is still unclear, though galactic mergers are thought to be involved. There is no evidence of anything like this ever having happened in ESO 320-G030.
“It is possible, but we do not still know, that many bulges were formed like that in ESO 320-G030, with intense nuclear bursts of star formation accompanied by quick growth of nuclear supermassive black holes,” Gonzalez-Alfonso said. “Therefore, it is imperative to observe these faraway galaxies in lines of H2O and other molecules with lines in the far-IR that would indicate such extreme events, in order to see their overall relevance in galaxy evolution across cosmic times.”
The truth about these galaxies must be out there, and somewhere far, far out there.