Create a free profile to get unlimited access to exclusive videos, sweepstakes, and more!
How did the COW supernova get so bright so fast? A little pre-explosion gas.
A superluminous supernova lit up previously ejected material.
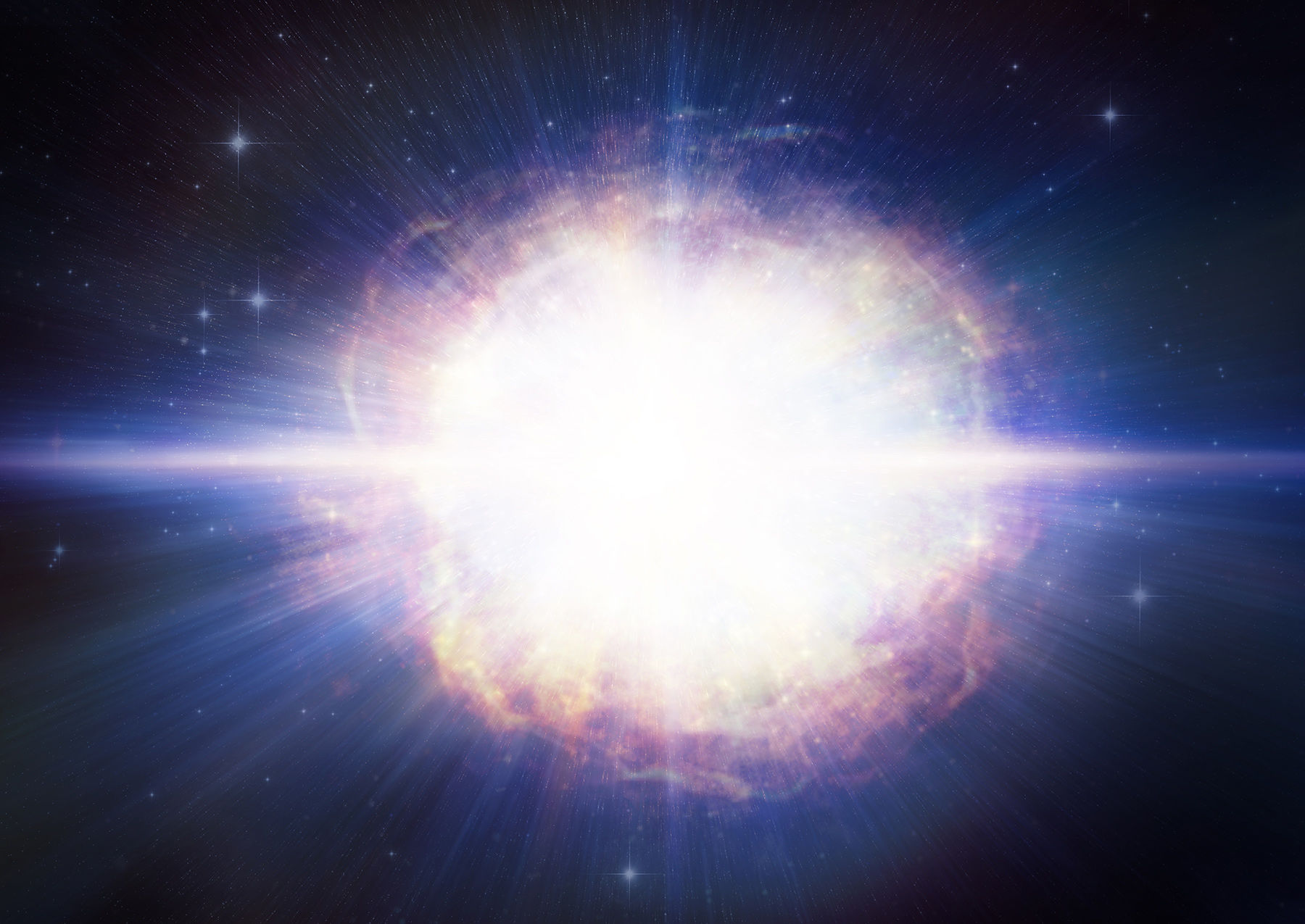
When a massive star dies at the end of its life, the core collapses to form either a very dense neutron star or a black hole. At the same time, the outer layers of the star are explosively launched into space by the colossal energy generated in the core collapse. The blast wave is so powerful that the expanding debris lights up with as much energy as billions upon billions of times the energy emitted by the Sun, and in fact can outshine an entire galaxy.
A supernova is born. But not all supernovae are created equal.
Some are far more powerful than others. The description above is ridiculously oversimplified — here's a somewhat more detail explanation, and more technical ones can easily be found online — and changing conditions from star to star can change the way one explodes. It can depend on things like having a binary star companion or not, the mass of the star when it explodes, the mass it had when it was born, how much of its outer layers blow off before the explosion, and more.
Still, there are supernovae that are exceptional even in this bewildering variety of explosions, and they can be very hard to explain. One in particular stands out, and astronomers think they can now explain what happened.
On June 16, 2018, the Asteroid Terrestrial-impact Last Alert System detected a supernova in a galaxy roughly 200 million light years away. The naming convention for these events dubbed it AT2018cow, and because astronomers are dorks just like everyone else it was known thereafter as the COW.
Other observatories were alerted and started watching it as well, and as the data came in astronomers were surprised to see just how luminous this explosion was — 100 billion times brighter than the Sun! This puts it in the rare class of superluminous supernovae, ones which are far more powerful than average.
But it also acted weirdly. It rose in brightness much faster than usual as well. Most supernovae take about two weeks before hitting peak luminosity, but this one rose in brightness by a factor of 100 in a single day. It also faded much more rapidly than typical supernovae as well. The COW's color in early days was blue, so this new type of event was called a Fast Blue Optical Transient, or FBOT. I wrote a synopsis of all this not long after it happened, if you want the details.
In the years since there has been a lot of work trying to figure out how to make a star explode like this, with limited success. Ideas have included the birth of a super-magnetized neutron star called a magnetar, or a white dwarf torn apart by a black hole (!!), and more. But now a team of astronomers thinks they have the answer (link to paper).
Sometimes, in the millennia or even years before the final explosion, massive stars can blow a lot of their outer layers away. Sometimes it's slow — Betelgeuse, for example, blows out a slow dense wind of matter — and sometimes it's more violent. This material surrounds the pre-supernova star so it's called the circumstellar matter.
We have physical models for how the interiors of such stars behave, equations that can be solved to understand what's happening deep inside a star. These can then be used to see what happens when the star explodes, and try to match the energies emitted to what's actually observed.
The astronomers modeled the COW as a very massive star, one born with a whopping 80 times the mass of the Sun. That's huge, and it's very rare for stars to get this big. Such a star loses much of its hydrogen outer layers during its life, blowing them away to great distance.
What's left is a still-crushingly-hefty core 42 times the Sun's mass that's mostly helium. At this point the star's core is fusing ever-heavier elements in its very center, layered like an onion. Some of that helium on the surface is blown into space as well, and the best model fit to the observations indicates about half a solar mass was surrounding the core when it blew up. That's less than in some cases, but still about 150,000 times the mass of the Earth.
The core is so massive a very bad thing happened deep inside it. When it started fusing oxygen into silicon the reaction is so energetic it produces super-high-energy gamma rays, the highest energy form of light. These gamma rays in part support the core against its own immense gravity, heating the interior enough to keep it inflated.
But gamma rays at these energies are unstable. They can spontaneously convert themselves into matter, an electron and anti-electron, a process called pair production. This steals away energy supporting the core, so gravity squeezes it, making it smaller. The temperature goes up, the core expands a bit, and finds equilibrium again. Until, that is, gamma rays start converting again, and the process repeats. This causes the core to pulsate, which helps it blow material off its surface.
At this point its life can now be measured in days, maybe weeks. Helium blows off its surface, and the pulsations get bigger and bigger, until finally — as it always does in the end — gravity wins. The core collapses, the temperature screams way up, and it explodes.
The total energy released in just a few days is truly staggering, as much as the Sun gives off over its entire 10 billion year lifetime. The blast wave from this titanic explosion slams into the previously ejected helium, lighting it up. If there had been a lot of this material around the star, more than the mass of the Sun, it would've taken many days to light it up and even longer for it to fade. But because it was only half a solar mass it brightened and faded rapidly.
The astronomers find that their model reproduces that rapid change in brightness of the first 20 days of the supernova pretty well. After that, the usual sort of supernova models work, with about 0.6 solar masses of radioactive nickel created in the nuclear furnace decaying into cobalt, generating so much light it explains the supernova's brightness thereafter.
Interestingly, they find that the total energy of the explosion doesn't affect the brightness over time — what astronomers call a light curve — very much at all, but what really dominates here is the material around the star, its shape, and its density. That's the key to understanding these fast supernovae.
For truly massive stars, over 100 times the mass of the Sun, the core explosion is so violent it tears itself apart completely, leaving nothing behind. In this case it may have left a black hole or a magnetar, and material falling back on this object may explain the brightness many weeks after the explosion. But the first few weeks are dominated by the circumstellar matter.
Now that these models exist it'll be interesting to see how they apply to any future such FBOT supernovae. Fitting a specific case is one thing, but generalizing it to be able to explain others is a strong indication that they're doing something right.