Create a free profile to get unlimited access to exclusive videos, sweepstakes, and more!
Wait. *How* big are neutron stars again?
Bottom line: Neutron star sizes are hard to figure out.
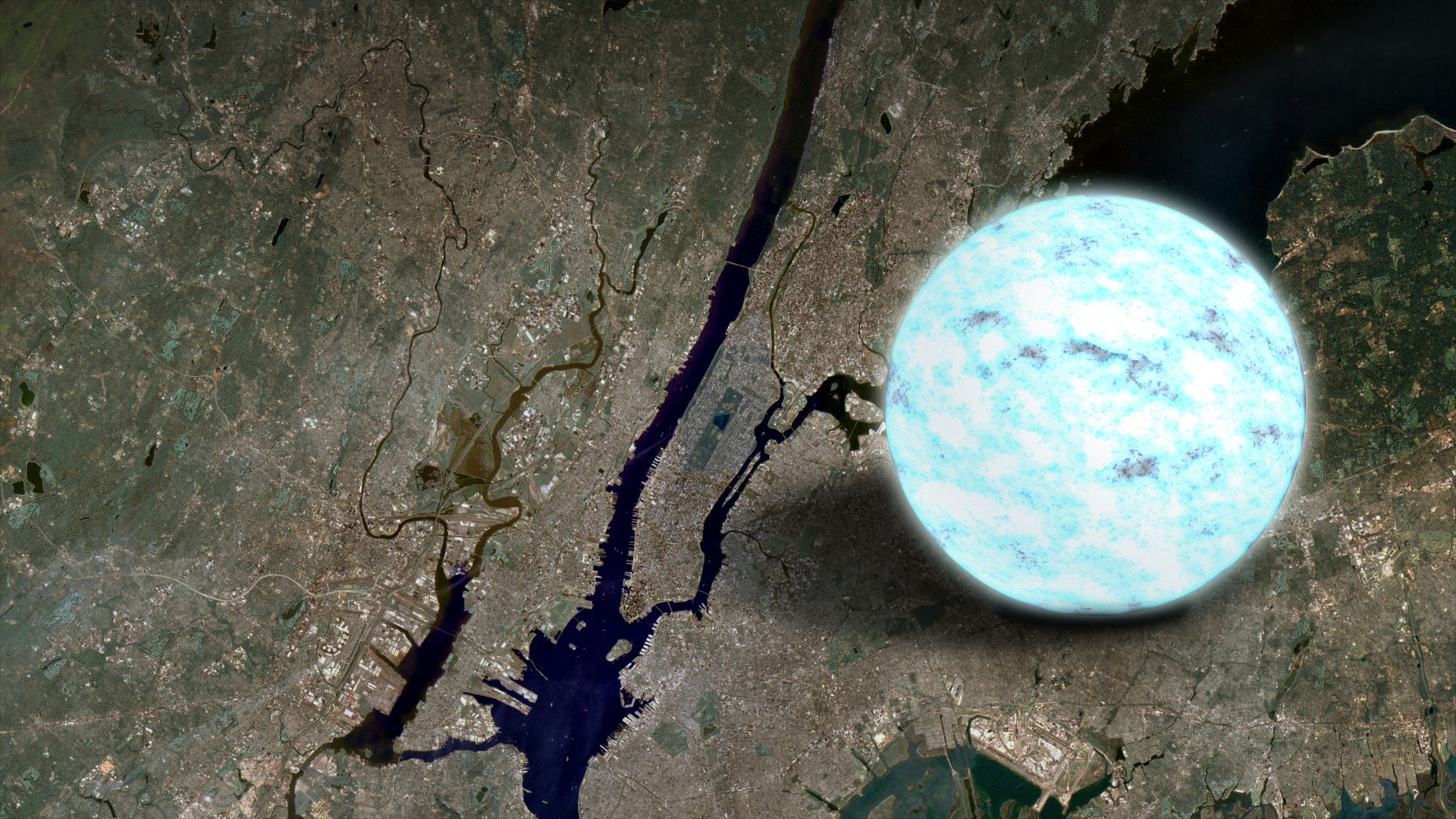
Neutron stars are so weird.
They are the leftover cores of massive stars that explode as supernovae. When the core of the star runs out of useable nuclear fuel to fuse, it collapses. This sets up a complicated series of events that touches off an immense explosion that blasts away the stars outer layer, creating the supernova. But the core collapses, and if it’s less than about three times the mas of the Sun, all the protons and electrons (plus a soupçon of antineutrinos) will combine to form neutrons.
These can be packed very tightly together, and when you have a couple of octillion tons of them, they will form a ball a couple of dozen kilometers across.
That’s small. That much mass crunched down into that tiny a ball means the density is ridiculous, a couple of hundred million tons per cubic centimeter. That’s like taking every single car in America and crushing them down so they are compressed into a cube the side of a six-sided die. Try rolling that for initiative.
The gravity on the surface of a typical neutron star is billions of times Earth’s gravity. The magnetic field is so strong it can wipe a credit card stripe clean from a half million kilometers away.
And maybe the weirdest thing of all in a miasma of other weirdness is that we don’t really know how big they are.
That’s important. The density is strongly dependent on the exact size, and that controls a lot of their behavior, so not knowing the size is a pain. For a long time, the best we could do is say they’re roughly 10 - 15 kilometers in radius.
However, new research using a sophisticated combination of techniques has come to the conclusion that a typical neutron star — one with 1.4 times the mass of the Sun — would have a radius of 12.01 ±0.78 kilometers (for the stats nerds, that’s the 90% confidence level).
They needed a lot of different information to get to this number. For example, in 2017 the gravitational waves and flash of light from a pair of neutron stars colliding reached Earth. Called a kilonova, the details of the explosion depend on things like the sizes of the neutron stars that merged, so the observations help constrain the sizes. For another, there is a field of advanced physics, called quantum chromodynamics, which governs how the matter in a neutron star should behave, which can be used to calculate their sizes.
New to this work is also including actual results from high-energy particle accelerators, specifically the Schwerionen Synchrotron 18 accelerator, which can send gold nuclei slamming into each other at a mind-flensing speed of 90% that of light. When that happens, the nuclei undergo pressure conditions similar to that inside a neutron star shortly before shattering into a zillion subatomic particles. The results of that shattering yield critical insight on the conditions of the nuclei during the collision.
Putting all this together, they get that 12.01 kilometer radius figure [link to paper]. So what does this mean?
Well, a similar calculation (minus the gold vaporizing) was done recently, and got a slightly smaller number: 11.0 ±0.9/0.6 km (again at the 90% level). Those might seem very different, but they’re not, really. Note the uncertainties, the ± numbers. The new value could be as low as 12.01 – 0.78 = 11.23, and the older value could be as high as 11.0 + 0.9 = 11.9 kilometers. Those overlap quite a bit, so we say they’re consistent with each other. If the uncertainties were lower we might have an issue, but while the two values are different they’re not wildly different.
But that difference is about 8%, and if accepted at face value that does change things. For example, if the neutron star is smaller it’s denser, and its gravity is stronger. That changes what happens when two of them collide; they can get much closer together before disrupting one another.
At the smaller size a neutron star won’t get ripped apart even by a black hole. That’s a very big deal indeed: That means the black hole swallows the neutron star whole. If instead the neutron star were bigger and got ripped apart there would be a very, very bright flash of light as that happened, something we could hope to see with a telescope, which would be a big breakthrough. But if the neutron star just gets et, bloop, we see nothing. In both cases the event would emit a blast of gravitational waves, but it would do so differently, and seeing the light emitted would help nail down a lot of the properties of the system.
So these predictions matter, and have real-world consequences. Well, real-Universe ones, at least for astronomers. And hey, we’re trying to understand how the Universe works here. Neutron star collisions produce elements like strontium, platinum, and gold, which are valued by humans. Figuring out how they came to be is at least interesting, and at best helps us unlock more secrets of the cosmos.
So yes, getting the sizes of neutron stars right has far-reaching ramifications. But we’re zeroing in on it, and as more kilonovae are seen, and more gold atoms are smashed, we’ll get even closer.